Schematic representation of the olfactory bulb network. Modified from Nagayama, Homma, and Imamura 2014.
When we think about how we use our senses to interact with the surrounding environment, the sense of smell might not be the first to come to mind. However, evidence indicates that olfaction plays a crucial role in our species, influencing several aspects of our social interactions, such as mate choice and mother-infant bonding (Boesveldt and Parma 2021).
One study estimated that humans can discriminate more than a trillion different odors (Bushdid et al. 2014). This remarkable capability is due to the presence of a large number of olfactory sensory neurons in the back of our nasal cavity. Each neuron expresses a single type of olfactory receptor protein, which has a distinct affinity for specific odorant molecules. The axons of olfactory sensory neurons that encode the same type of receptors converge in one or two discrete subregions at the surface of the olfactory bulb (OB), known as glomeruli. The sensory information from different glomeruli is then integrated by various cell types deeper in the OB and sent to cortical and subcortical areas of the brain, enabling the perceptual discrimination of different odors (Nagayama, Homma, and Imamura 2014).
The mouse olfactory bulb
In mice, the olfactory bulb has been used to investigate various aspects of brain function, including the mechanisms underlying sensory processing and changes in OB structure and function due to disease or sensory deprivation. Like the neocortex, the dorsal part of the mouse olfactory bulb (dOB) is visible from above and can be examined using optical imaging (Leong and Storace 2024). Several studies have characterized the responses to odours using various optical imaging approaches, including intrinsic imaging (Chery et al. 2011), calcium imaging (Fried, Fuss, and Korsching 2002), and voltage-sensitive probes (Leong and Storace 2024). It is estimated that the mouse dOB contains more than a hundred glomeruli, which are clearly visible at a mesoscopic scale (Leong and Storace 2024). In this blog, we demonstrate the application of mesoscopic calcium imaging to assess the neuronal responses of the mouse dOB to distinct odorants.
The experiment
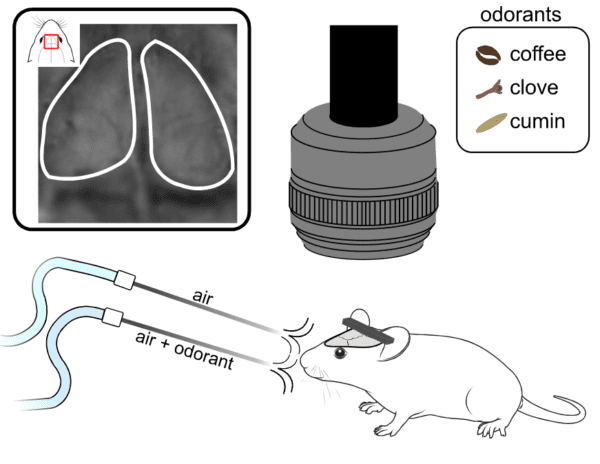
In this experiment, we recorded the neuronal activity from the dOB of an anaesthetized thy-1 jrGECO-expressing mouse. Cortical activity was captured using the Light Track OiS200 system with lime LED illumination (565 nm) at a frame rate of 20 Hz.
The odour stimulation was delivered using an air pump attached to a compartment where the odorant samples were added. We used three natural odorants: coffee, cloves, and cumin. Each stimulation trial consisted of delivering the odorant/air mixture to the animal’s nostrils for 3 seconds with 5 seconds of pure air (washout). Each odorant stimulation trial was repeated 10 times.
Calcium Imaging data analysis
The calcium signals were normalized so the pixel values are expressed as ΔF/F. Then, the data was separated by events and averaged. Cortical activation maps were generated by calculating the average of the responses between 0.2 and 1 second post-stimulus onset. For the glomeruli analysis, ROIs were created by segmenting the activation maps using a local adaptive threshold method.
Results
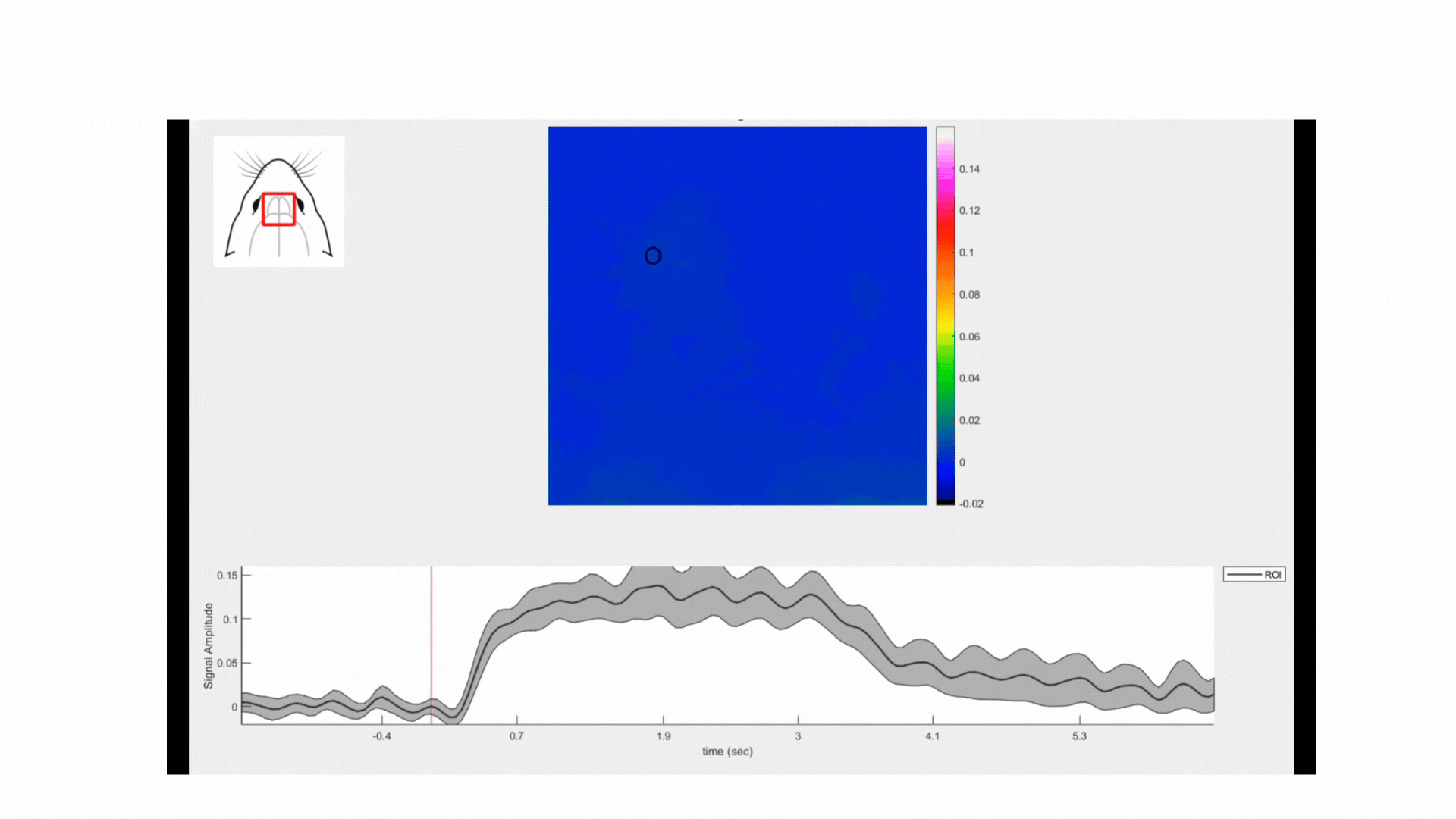
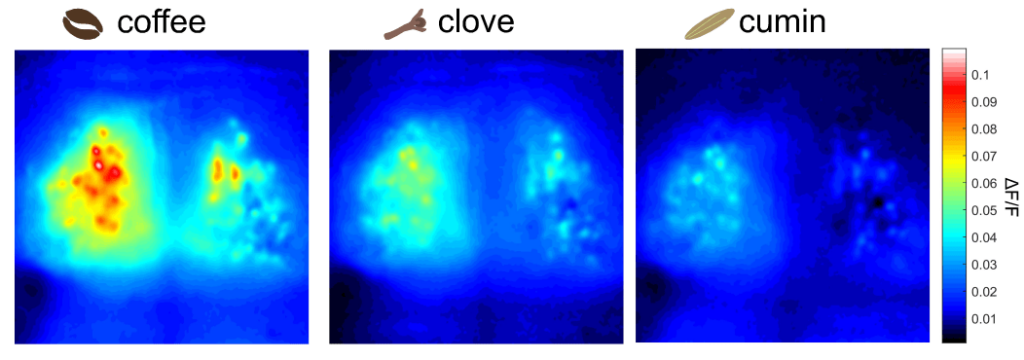
All three odorants used in this experiment elicited reliable responses in the mouse dOBs. For instance, in the movie below, one can observe the neuronal responses in the dOB following the inhalation of coffee scent. Note the oscillations of the calcium signal corresponding to the respiratory rate. Coffee was the odorant that yielded the strongest responses, followed by clove and cumin (see figure below).
Distinct Odorants activated different groups of glomeruli
In all recordings, a large number of glomeruli were clearly identified as these regions elicited the strongest responses to the odorants. This allowed us to delineate several glomeruli and assess their selectivity to each odorant. Interestingly, each stimulus preferentially activated distinct groups of glomeruli with varying degrees of selectivity (figure below).
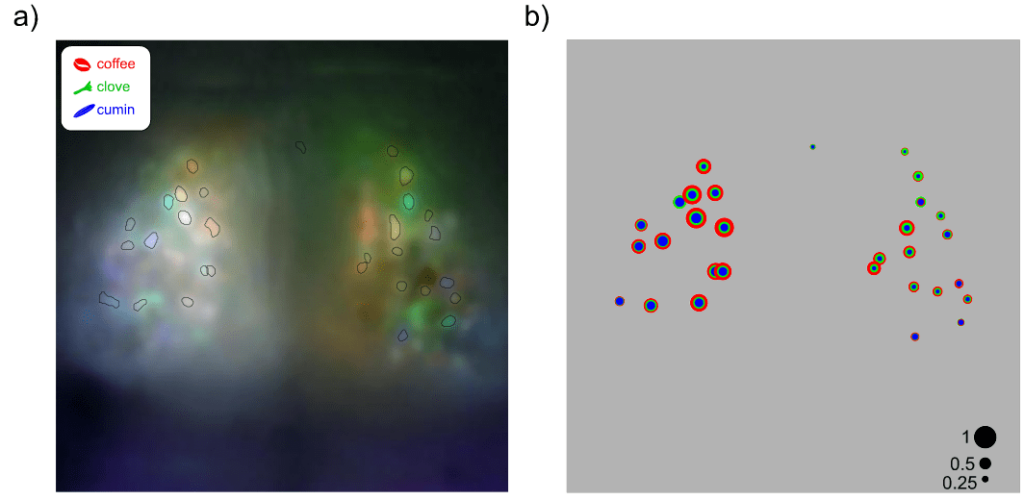
Summary and insights
This experiment showcases the use of mesoscopic calcium imaging to assess the function of the mouse olfactory bulb. One of the key findings is that the glomeruli within the dOB can be easily delineated at mesoscopic resolution, allowing for a detailed assessment of their responses to different odorants. The ability to distinctly visualize and measure glomerular activity makes mesoscopic imaging a valuable tool for investigating the functional dynamics of the olfactory bulb.
Products used
References
Boesveldt, Sanne, and Valentina Parma. 2021. “The Importance of the Olfactory System in Human Well-Being, through Nutrition and Social Behavior.” Cell and Tissue Research 383 (1): 559–67. https://doi.org/10.1007/s00441-020-03367-7.
Bushdid, C., M. O. Magnasco, L. B. Vosshall, and A. Keller. 2014. “Humans Can Discriminate More than 1 Trillion Olfactory Stimuli.” Science 343 (6177): 1370–72. https://doi.org/10.1126/science.1249168.
Chery, Romain, Barbara L’Heureux, Mounir Bendahmane, Rémi Renaud, Claire Martin, Frédéric Pain, and Hirac Gurden. 2011. “Imaging Odor-Evoked Activities in the Mouse Olfactory Bulb Using Optical Reflectance and Autofluorescence Signals.” Journal of Visualized Experiments (JoVE), no. 56 (October), e3336. https://doi.org/10.3791/3336.
Fried, Hans U., Stefan H. Fuss, and Sigrun I. Korsching. 2002. “Selective Imaging of Presynaptic Activity in the Mouse Olfactory Bulb Shows Concentration and Structure Dependence of Odor Responses in Identified Glomeruli.” Proceedings of the National Academy of Sciences 99 (5): 3222–27. https://doi.org/10.1073/pnas.052658399.
Leong, Lee Min, and Douglas A. Storace. 2024. “Imaging Different Cell Populations in the Mouse Olfactory Bulb Using the Genetically Encoded Voltage Indicator ArcLight.” Neurophotonics 11 (3): 033402. https://doi.org/10.1117/1.NPh.11.3.033402.
Nagayama, Shin, Ryota Homma, and Fumiaki Imamura. 2014. “Neuronal Organization of Olfactory Bulb Circuits.” Frontiers in Neural Circuits 8 (September). https://doi.org/10.3389/fncir.2014.00098.
Drawings used:
https://doi.org/10.5281/zenodo.3925901
https://doi.org/10.5281/zenodo.3925903